Editor's Note: In 1755, a great earthquake devastated Lisbon, killing tens of thousands of people and destroying or rendering uninhabitable most of the wealthy city's buildings. Because most of the effects of the offshore quake were caused by the massive tsunami and widespread fires that followed, rather than by ground shaking, it was believed that modern construction and response systems would mitigate much of the damage were such an event to recur today. Recent seismic hazard studies, however, suggest that an onshore quake capable of causing significant damage in Lisbon is much more likely than previously contemplated. Dr. Guillermo Franco, AIR Senior Engineer, and Dr. Bingming Shen-Tu, Principal Scientist, Seismology, discuss the implications.
The 1755 Lisbon Earthquake
The Great Lisbon Earthquake occurred in the morning hours of All Saints Day, a Catholic high holiday, on November 1, 1755. Much of the city's population, estimated to be around 200,000, was gathered for mass in Lisbon's opulent churches and cathedrals. Beginning at around 9:30 am, three separate jolts spaced a few minutes apart violently shook southwestern Portugal.
Those who survived collapsing buildings fled into the streets, leaving behind lighted candles on church altars and cooking fires in kitchens. Intense fires that were likely ignited by curtains and woodwork that fell into the flames spread within minutes throughout the city and burned out of control for days.
Some residents sought refuge in the harbor at the mouth of the Tagus River, but their relief from the fires and falling debris was short-lived. Approximately half an hour after the first shock, massive tsunami waves up to 12 meters in height began to arrive, wrecking ships and drowning thousands of people. Towns along Portugal's western and southern coasts, southwestern Spain and most of Morocco's Atlantic coast also suffered significant damage and loss of life. The effects of the tsunami were noted as far away as England to the north and in the Caribbean islands across the ocean.
According to a 2003 study by Alvaro Pereira, nearly 70% of Lisbon's dwellings—about 23,000 buildings—were destroyed or substantially damaged by the combined effects of shaking, fire and tsunami.1 In total, Pereira estimates losses of 64,000 to 72,000 contos, about 32% to 48% of Portugal's GDP at the time.
This article describes the tectonic processes that gave rise to the disaster that occurred some 250 years ago. It also addresses recent research that suggests that Lisbon's earthquake risk today is driven not by the prospect of a recurrence of a 1755-type event—which is estimated to have a return period of between 3,000 and 4,000 years—but rather by the possibility of an earthquake of more moderate magnitude, but one much closer to the capital. As it turns out, that more likely scenario has the potential to produce losses as high, or even higher than the occurrence of a much larger earthquake offshore.
Seismotectonic Setting
The 1755 Lisbon earthquake, with a magnitude estimated at 8.5 to 9, is the largest known historic earthquake to impact Europe and northern Africa. Various studies2 have located the epicenter at about 300 km to 400 km southwest of Lisbon in the Gorringe Bank, along the Africa-Eurasia plate boundary.
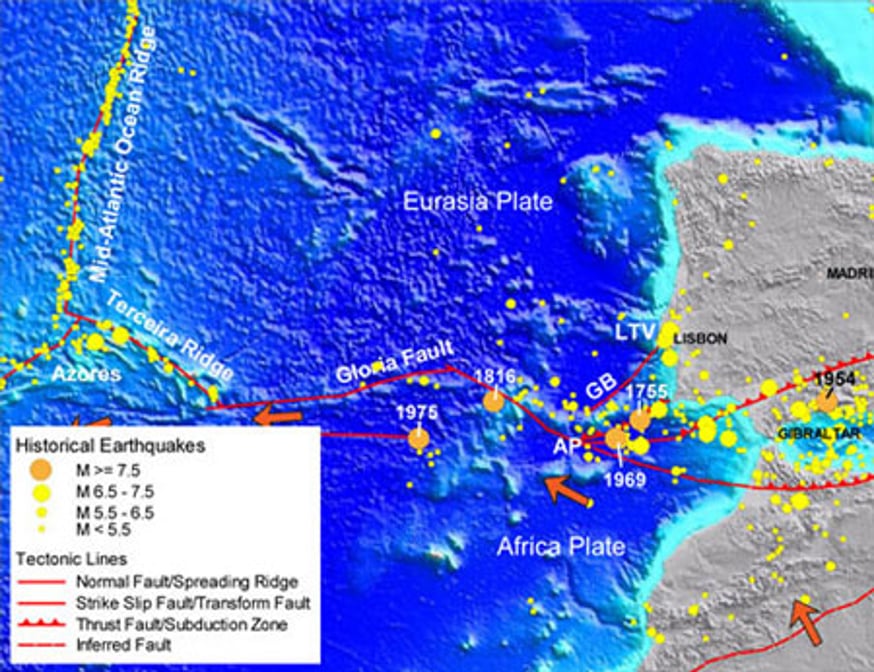
Figure 1. Active tectonics and historic seismicity in the 1755 Lisbon earthquake source zone (GB = Gorringe Bank, AP = Abyssal Plain, LTV = Lower Tagus Valley; the arrows indicate the rotation of the Africa Plate). Source: AIR
The Africa-Eurasia plate boundary extends from the mid-Atlantic ocean ridge triple junction near the Azores eastward to the Strait of Gibraltar. Here the Africa plate rotates counterclockwise relative to the Eurasia plate at a rate of about 4 mm/yr. That's relatively slow compared to other active plate boundaries. Nevertheless, several large magnitude earthquakes have occurred here, including the 1755 event, a M7.6 earthquake in 1816, and a M7.9 earthquake in 1969.
This plate movement has caused various types of deformation: extension along the Terceira Ridge around the Azores Islands in the west; strike-slip along the Gloria Fault in the central segment; and convergence and collision in the Gorringe Bank and the Strait of Gibraltar to the east.
In the west and central segments, the Africa-Eurasia plate boundary is a well defined narrow fault zone. East of the Gloria Fault, however, it becomes a diffusive collision zone between two continents and continental shelves. The active deforming belt is about 200 km in the Gorringe Bank and widens to more than 500 km east of Gibraltar.
The collision stress not only loads up major structures within the collision zone, where great earthquakes including the 1755 Lisbon earthquake originated, but also many onshore crustal faults at the Iberia and Africa continent margins. Several onshore faults in southwestern Portugal have been found to be seismically active. Most notable is the active fault zone in the Lower Tagus Valley (LTV). This is this zone that has gained considerable attention in recent years.3 The reason is simple: it passes through Lisbon, Portugal's most populous city.
Several large historical earthquakes, including the 1531 M7, the 1909 M6.3 and possibly the 1344 M>6, occurred on the LTV, causing significant damage in the Lisbon area. Furthermore, one study4 proposed that the offshore 1755 earthquake actually triggered an onshore rupture on the LTV fault. Thus the return period of magnitude 6 to 7 earthquakes along the LTV could be as short as 150 to 200 years, making Lisbon the highest risk area in Portugal.
Importantly, from a risk assessment perspective, an earthquake of magnitude 6 to 7 close to Lisbon could cause comparable or even greater damage than a stronger shock offshore. For example, the 1531 M7 earthquake produced a higher intensity in Lisbon than the 1969 M7.9 Abyssal earthquake, and similar to the 1755 Lisbon earthquake.
Vulnerability of Lisbon's Building Stock
How prepared is Lisbon's building stock? Typical of many European countries, reinforced concrete is the predominant type of construction in areas of Portugal urbanized in the last few decades, accounting for roughly 50% of the current building inventory. Historic districts and city centers, however, contain masonry structures that may be up to several hundred years old. Portugal's masonry construction, which accounts for some 36% of the building stock, displays a wide range of building techniques and construction quality. A significantly smaller percentage of the building stock—around 12%—is steel construction, mainly consisting of industrial and commercial structures. Finally, there remain traces of traditional timber construction, although the fraction of such buildings is very small.
Masonry buildings in Lisbon exhibit a variety of typologies, ranging from the traditional rubble-stone double-wythe two- or three-story building to the more sophisticated "pombalino" structures, characterized by a braced timber frame with masonry infill. One study5 found that the poor maintenance of aged masonry structures in Portugal—some over 200 years old—may have a significant impact on their vulnerability. Mortar decays over time, and failure to monitor and maintain this bonding layer in masonry walls can increase the building's vulnerability. The conclusion was that the masonry buildings in Lisbon's historic districts might therefore be somewhat more vulnerable than buildings of a similar age in other parts of Europe.
It should be said, however, that much of Portugal's historic unreinforced masonry construction does not qualify for coverage under typical earthquake policies. After excluding properties considered uninsurable, the predominance of reinforced concrete construction becomes even more pronounced, at 66% of the building stock (Figure 2).
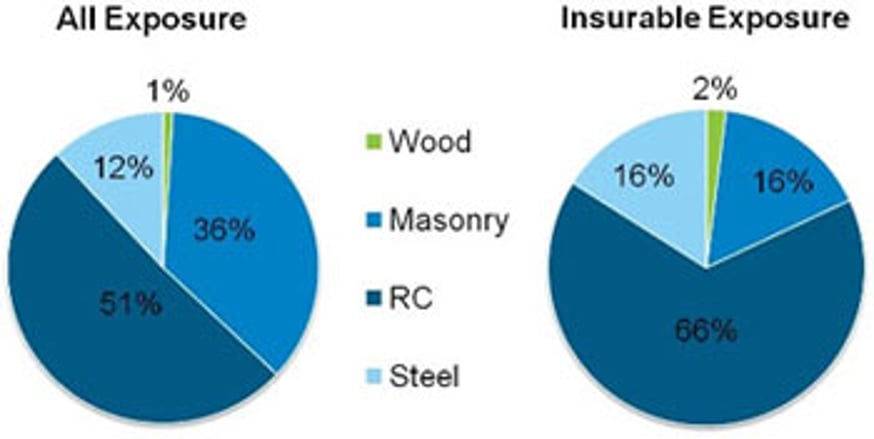
Figure 2. Portugal's building stock by construction material. Source: AIR
The vulnerability of reinforced concrete is greatly influenced by the building code. While the first seismic provisions in construction guidelines appeared in the literature after the 1755 Lisbon earthquake, they were not widely applied or enforced. Modern building code development was prompted by a strong earthquake in the Lower Tagus Valley in 1909 that devastated the town of Benavente, which resulted in the publication of the first seismic code in 1958.
In 1983 a new code, Regulamento de Seguranca e Accoes para Estruturas de Edificios e Pontes, was published, followed by two other important codes for reinforced and pre-stressed concrete structures and for steel structures. The 1983 code divided Portugal into four seismic zones (Figure 3) according to historical seismicity, and will remain in effect until the transition to the national implementation of Euro Code 8 takes place in full.
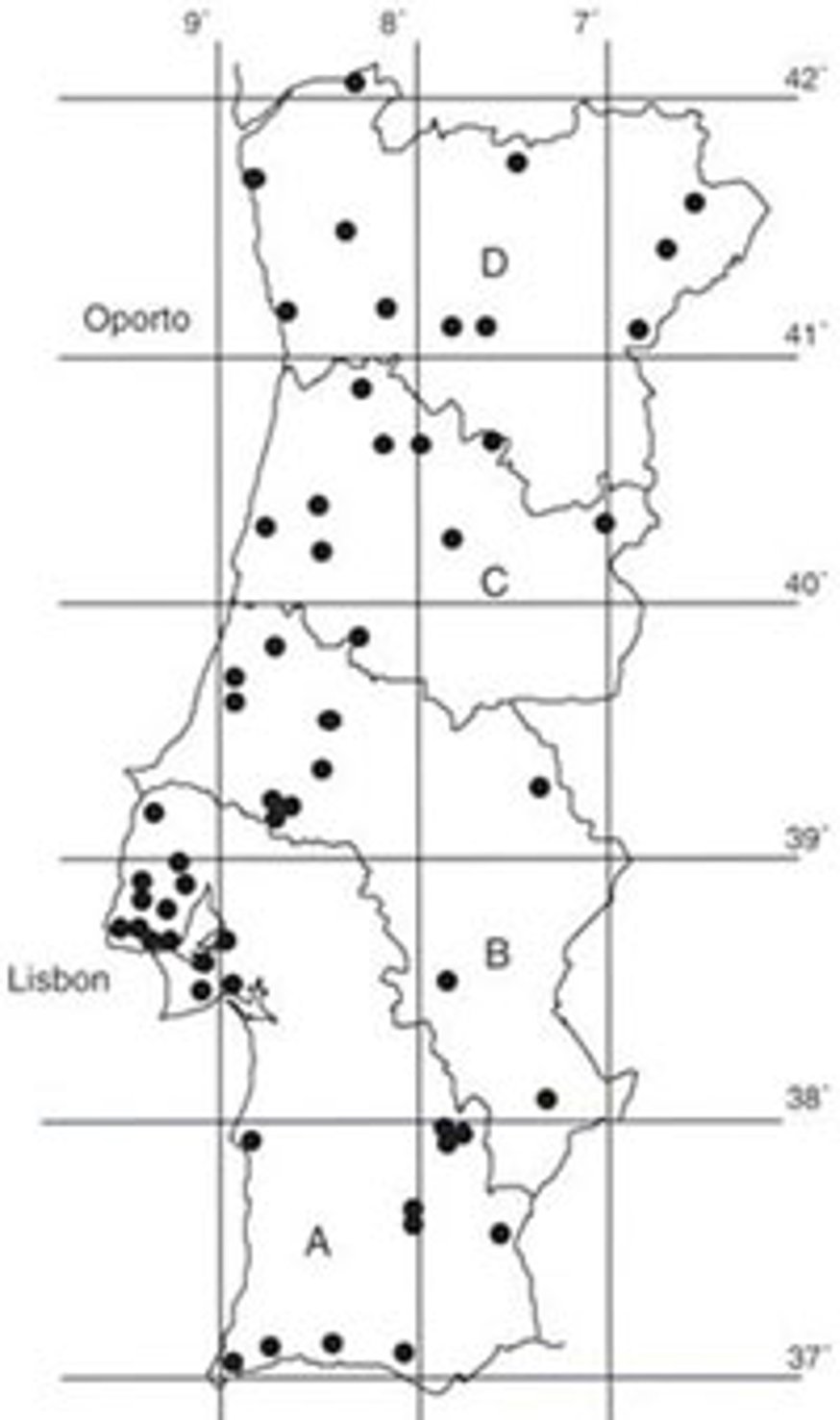
Figure 3. Building codes in Portugal use a seismic zonation based on expected ground motion; current code specifies four zones (from Paz 1994). Source: AIR
The zones were defined by the level of maximum expected peak ground acceleration. Zone A, the region with the highest level of seismic hazard, covers the southwest continental territory, including Lisbon, and several of the Azores islands just east of the Mid-Atlantic Ridge. Zones B, C, and D are located progressively further north along the Iberian Peninsula. Zone D includes the Madeira archipelago and the Flores and Corvo Islands, located on the North American Plate. More recent work carried out at Portugal's Laboratório Nacional de Engenharia Civil has resulted in new seismic loading zones to be implemented in Portugal's national annex to Euro Code 8. Seismic hazard is computed for both inter-plate and intra-plate events and the result is the zone classification shown in Figure 4. Note that for intra-plate, or onshore events like the one contemplated in the next section, Lisbon belongs to Portugal's highest seismic risk zone.
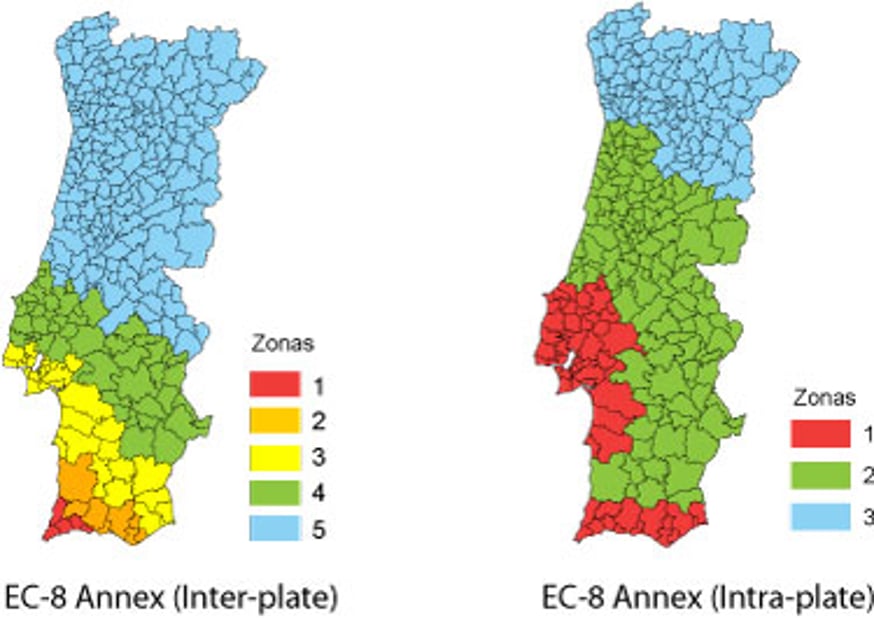
Figure 4. Euro Code 8 zones (Portugal Annex) for inter-plate and intra-plate seismicity (from Azevedo and Guerreiro, 2007). Source: AIR
A Modern Day Scenario
The somewhat higher-than-average vulnerability of the building stock, coupled with the high seismic potential of crustal faults in the Lower Tagus Valley, can generate large loss scenarios in the Lisbon area. Indeed, although earthquake risk in Lisbon is perhaps most often associated with a recurrence of an event similar to the 1755 offshore rupture—which AIR estimates would result in ground-up earthquake shake losses of 12 billion Euros6—the stochastic catalog of Version 11.0 of AIR's Mediterranean earthquake model includes several costlier scenarios that originate from the nearer onshore LTV source zone.
One such event is a magnitude 6.6 earthquake originating from a source very near the historical 1344 earthquake. AIR estimates that with a focal depth of just over 17 km, this event would result in ground-up losses (before the application of insurance terms) of nearly 20 billion Euros. Note, again, that this figure reflects shake damage to insurable buildings and contents only.
Figure 5 shows the simulated geographic distribution of losses, which include shake-induced building and contents damage to insurable residential, commercial, and industrial properties.
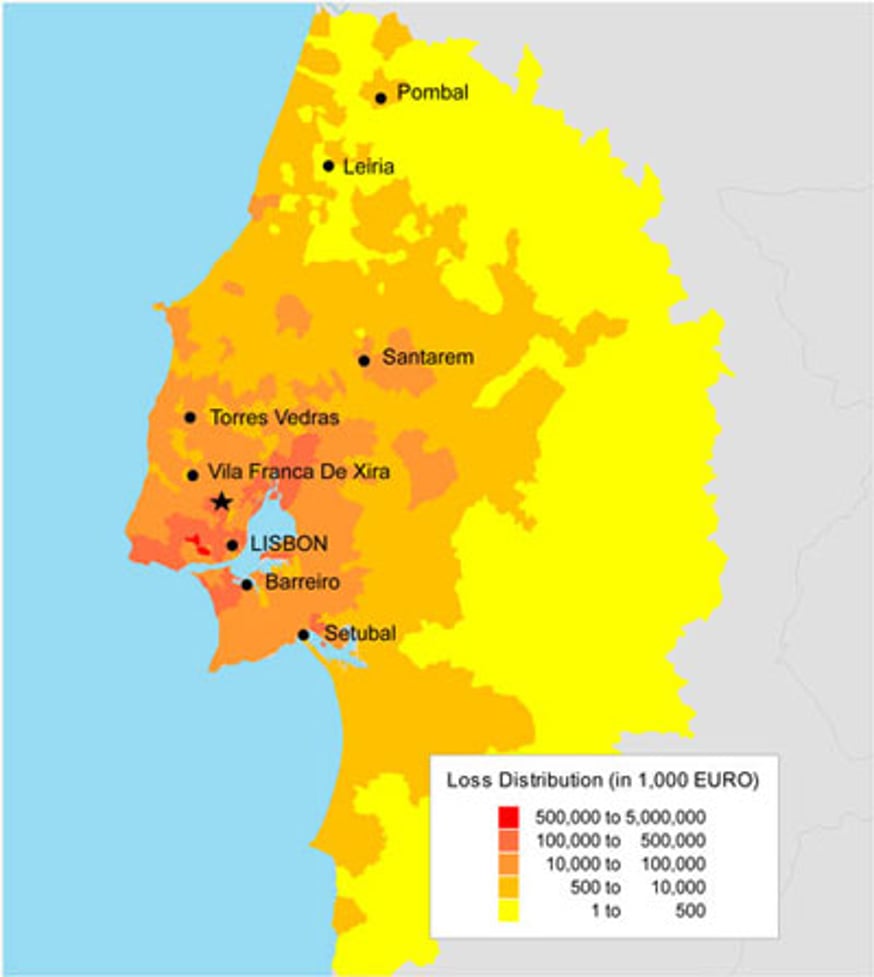
Figure 5. Geographical distribution of insurable losses (the star marks the epicenter of the simulated M6.6 event). Source: AIR
In simulating this event, three construction types—unreinforced masonry, reinforced concrete with moment resisting frame, and reinforced concrete with unreinforced masonry infill walls—were analyzed to determine their likely performance.7
Not surprisingly, unreinforced masonry, or URM—typically constructed prior to the 1940s—perform poorly. As shown in Figure 6, a large area of several hundred square kilometers, including the city of Lisbon, is expected to sustain a mean damage ratio (ratio of the repair cost to the total replacement value of the building) of 15% or more to URM construction.8
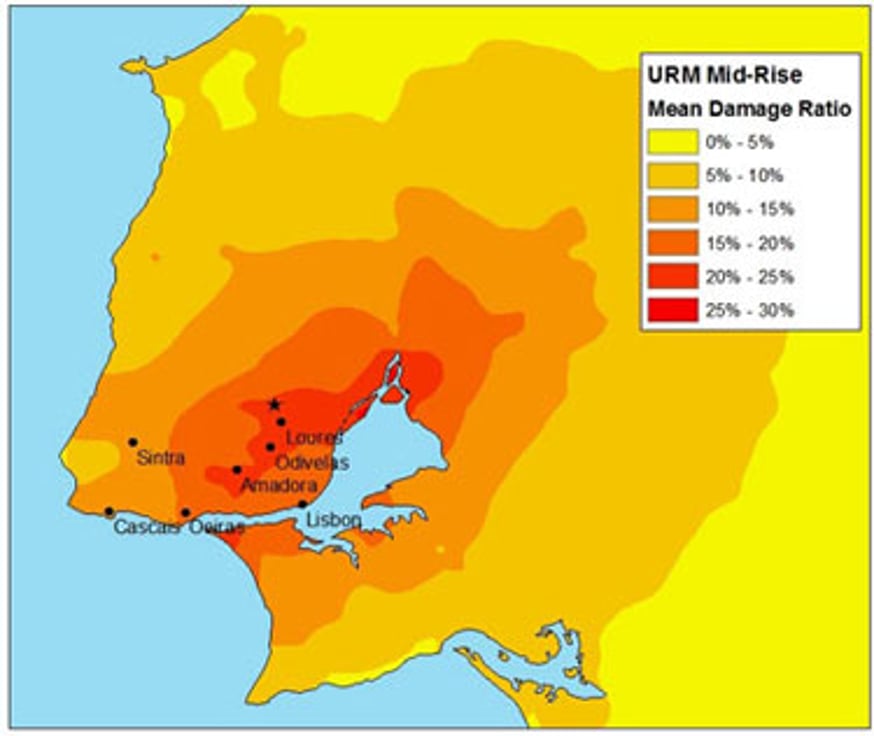
Reinforced concrete structures with moment resisting frames perform significantly better in this analysis—with a mean damage ratio of 5% to 10% (see Figure 7). These structures, mostly constructed after the 1960s, use a system of beams and columns to support lateral loads.
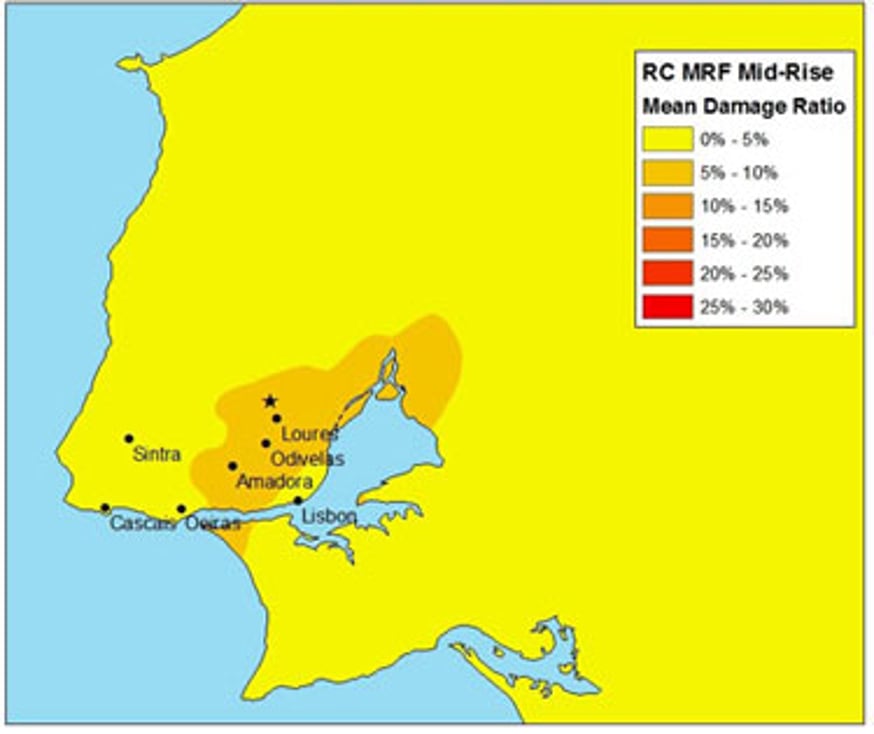
Figure 7. Mean damage ratio of reinforced concrete with moment resisting frame. Source: AIR
In some instances, reinforced concrete is built poorly and the frame of beams and columns is insufficient to prevent severe lateral deformation of the building. In those cases, the infill walls made of masonry start playing a structural role. This behavior has been observed in several events across Europe and is accounted for in the AIR model. Typically weaker than a well-designed reinforced concrete frame, this class—reinforced concrete with URM walls—is expected to perform somewhere in between masonry classes and reinforced concrete classes, depending on height and age. Figure 8 shows the results obtained for this typology.
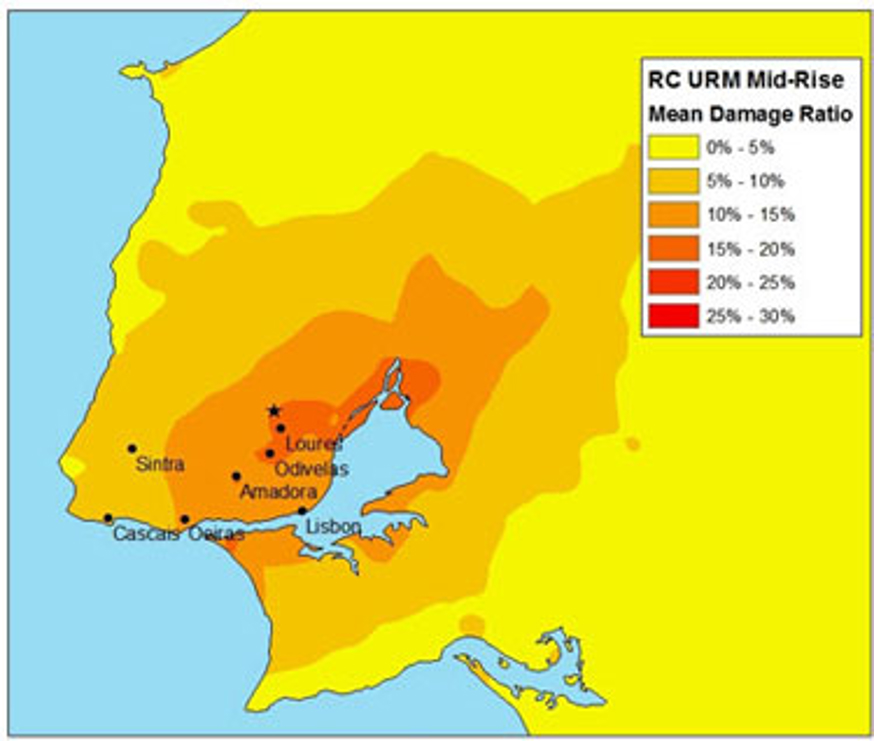
Figure 8. Mean damage ratio of reinforced concrete with unreinforced masonry infill walls. Source: AIR
Conclusion
The 1755 earthquake in Lisbon required an unprecedented response and reconstruction effort, giving rise to its status as the first "modern disaster." The event spurred scholars, scientists and theologians to speculate on the origins—be it natural or divine—of the apocalyptic event, and the debate raged on for years in the works of prominent thinkers like Voltaire, Rousseau, and Kant. Ideology aside, the Lisbon earthquake brought about some of the first provisions for urban disaster mitigation and earthquake resistant building design. The event also prompted a widespread effort to investigate and record the effects of the earthquake throughout the affected area—giving rise to what some consider the birth of modern seismology.
Seismologists believe that the recurrence potential of a similar offshore earthquake is relatively low. Were a similar event to occur today, modern tsunami warning systems and disaster response practices, as well as superior building construction, would moderate the scale of damage and casualty. The more critical source of concern is the Lower Tagus Valley region, which could produce a magnitude 6 to 7 earthquake with a return period as short as 150 to 200 years. This seismic source zone, with its proximity to Lisbon, the large number of old masonry buildings and a fraction of reinforced concrete frames designed with limited lateral resistance, presents the most significant potential for large loss earthquakes in Portugal.
1 Most of the losses were likely the result of tsunami and fire; shake damage—even from a magnitude 8.5 earthquake—would have been relatively limited given the epicenter's offshore location.
2 Moreira, 1989; Zittellini et al., 1999; Baptista et al., 2003
3 Vilanova et al. (2003), Cabral et al. (2004), Carvalho et al. (2006)
4 Vilanova et al. (2003)
5 D'Ayala et al. (1997)
6 The AIR estimate reflects insurable losses from earthquake shake only; it does not include the effects of tsunami or fire-following.
7 The AIR model's damage functions were validated and refined based on a review of recent vulnerability studies. Sources include a study by Rossetto and Elnashai (2003) that derived fragility curves for reinforced concrete structures in Europe based on damage reconnaissance data from 19 earthquakes, as well as research by Speranza et al. (2006) and Di Pasquale and Goretti (2001) that examined the behavior of reinforced concrete and masonry buildings.
8 A mean damage ratio of 15% should be interpreted to mean that, on average, most buildings will sustain less than 15% of damage but, on the other hand, some buildings may collapse. Since collapses are less likely, the mean value remains at 15%. Secondary uncertainty implemented in the AIR models accounts for this natural dispersion observed in actual earthquake events.
References
Azevedo, J and Guerreiro, L. (2007), "Current Status of Seismic Isolation and Energy Dissipation R&D and Applications for Buildings, Bridges and Viaducts, in Portugal" 10th World Conference on Seismic Isolation, Energy Dissipation and Active Vibrations, Control of Structures, Istanbul, Turkey, May 28-31, 2007.
D'Ayala, D., Spence R., Oliveira, C. and Pomonis, A. (1997), "Earthquake Loss Estimation for Europe's Historical Town Centres," Earthquake Spectra, 13 (4), pp. 773-793.
Di Pasquale, G. and Goretti, A. (2001) "Functional and Economic Building Vulnerability Observed in Recent Italian Earthquakes," X National Conf. Earthquake Eng., Potenza-Matera, 9-13.
Paz, M. (1994) "International Handbook of Earthquake Engineering: Codes, Programs, and examples," Chapman and Hall, Inc., NY, NY.
Pereira, Alvaro S. (2003), The Opportunity of a Disaster: The Economic Impact of the 1755 Lisbon Earthquake, Department of Economics, University of York.
Rossetto, T. and Elnashai, A. (2003), Derivation of vulnerability functions for European-type RC structures based on observational data. Engineering Structures, 25 (10).
Speranza, E., Goretti, A. and Dolce, M. (2006), "Historical Damage Data and Microzonation: An Application to 1930 Senigallia Earthquake," Proc. 1st ECEES, #1267, Geneva, Switzerland.
Vilanova, S. P., C. Nunes, and J. Fonseca (2003), Lisbon 1755: A Case of Triggered Onshore Rupture, Bulletin of the Seismologic Society of America, 93, 2056—2068.